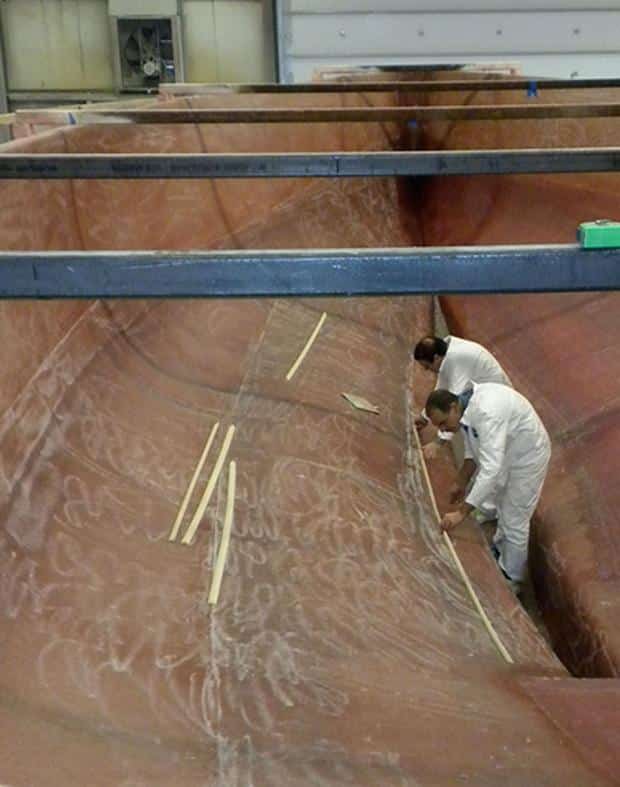
Most of today’s hulls and decks are built from marine “composites”—a combination of materials in which each part keeps some of its original traits. Broadly speaking, composite construction dates back several millennia, to an era when grass was mixed with mud to build a hut. in this first of two articles, we’ll explore the two basic components that go into today’s composite boats: the reinforcing fiber and the polymer matrix. As we begin, remember that a whole composite structure has properties all its own, and is more than the sum of its parts. Also, all composite structures depend as much on technique—how the materials were put together—as they do on the materials themselves.
Fiber Reinforcement
Modern composite boatbuilding needs fibers. Yes, composites start with polyester resin—the pour- able, sprayable thermoset- ting plastic that hardens after mixing with a chemical catalyst. if you tried to build a boat from polyester alone, you’d be able to create virtually any complex shape for virtually any hull form. in that regard, the material beats both wood and metal. in the 1940s, when engineers first started experimenting with boats made from thermosetting plastic, they loved the fact that they’d created entire hulls without any seams—no caulk, no welds. But with polyester alone you’d have an impossibly fragile boat.
In his excellent book Heart of Glass (International Marine, 2004), Dan Spurr tells how these materials were discovered and used. in the 1930s, researchers at Owens, Illinois Glass company tried to spin glass into fibers to replace mineral wool for insulation and filters. Almost accidentally, they revolutionized boatbuilding. Now, more than 90 percent of all boats are built from composites that started with their invention of fiberglass.
The glass fiber created in the 1930s is remarkably similar to today’s materials. the glass fiber exhibits astounding tensile strength and resists both stretching and contamination by chemicals. And, important for boatbuilders, the flexible fibers are relatively easy to work with.
Reinforcing Fibers |
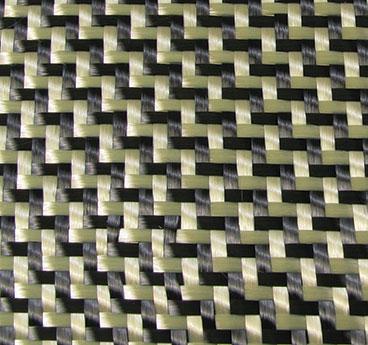
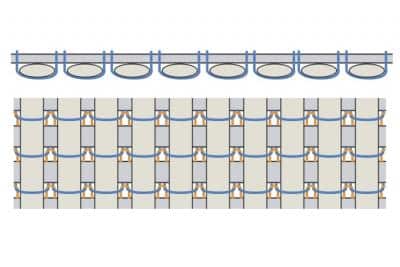
| |Through the 1970s and 1980s, boatbuilders grew much savvier about creating composite structures with the best strength-to-weight ratios. Knitted fabrics, which allow designers to engineer strength into composite structures along the lines of filaments, are one of the keys.| |
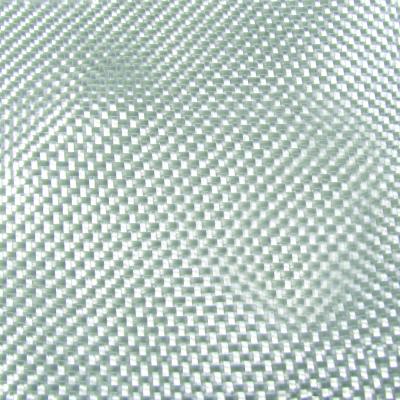
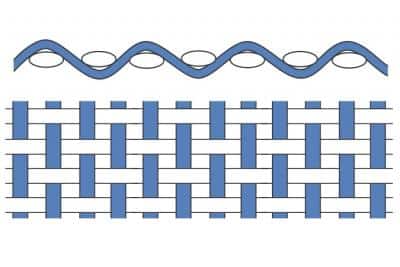
| |Woven roving was one of the great FRP (for fiber-reinforced polymer) boons of the 1950s. Many thousands of boats were built with this fabric technology. It allowed laminators to build up bulk in a composite structure very quickly. The downside is that its loose weave invites a heavier, resin-rich layup.| |
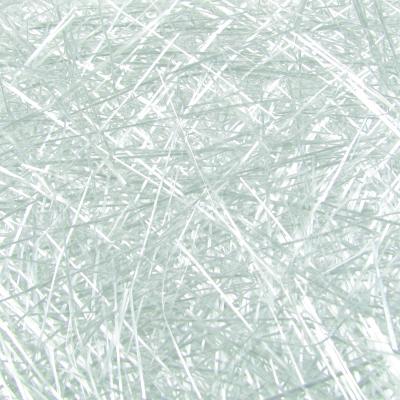
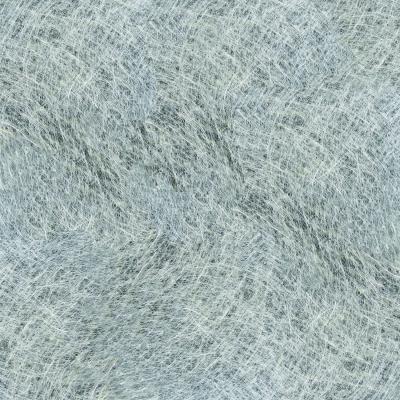
| |Fiberglass mat, composed of either chopped, or short, strands (above, left), or continuous long strands (above, right), is ideal for small FRP parts or as one layer in larger FRP parts. But mat alone provides comparatively little strength in a laminate.| |
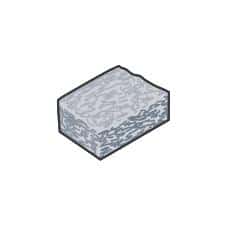
|
The silica-based fiberglass used in today’s boats comes in two varieties: E-glass and S-glass. E-glass is the workhorse of contemporary boatbuilding against which all others are measured. It delivers reasonable strength at relatively low cost. S-glass is stronger, lighter, and stretches less but costs three to four times more.
In the last 30 years or so, two new categories of fibers have changed the way high-performance boats are built: aramids and carbon fibers. Aramid stands for “aromatic polyamide.” Kevlar, the best-known aramid, is the trademarked name for a fiber that DuPont (the company that created polyester resin in the 1940s) originally developed for tires. Trade names for aramids from other companies include Technora, Twaron, and Heracron. Twice the tensile strength of E-glass; low weight; weavability; resistance to puncture, impact, and fatigue—these are the main virtues of aramid fibers. But they absorb water and don’t stand up to compression loads as well as glass fiber. For these reasons, they’re usually used in combination with other fibers.
For light weight and stiffness, the material to beat is carbon fiber. Its modulus of elasticity is roughly six times stiffer than E-glass. That stiffness, plus the fact that it doesn’t corrode like aluminum or stainless steel, makes carbon fiber ideal for such high-load components as masts and rudderstocks, and even for entire boats. But carbon-fiber parts need to be engineered carefully; carbon’s lack of stretchiness provides little warning before it fails, suddenly and catastrophically. It’s also by far the most expensive reinforcing fiber.
Think of reinforcing fibers in three dimensions: lines, planes, and cubes. Builders don’t use just fibers and filaments; they work with two-dimensional sheets of fabric called mat and woven roving and knits. The properties of a composite structure depend on both the fiber’s material and its orientation. Yacht designers can engineer each fabric layer, or ply, to provide optimal strength in a particular direction. Together, the three-dimensional layers in a composite structure are called the laminate.
In the first dimension, we need to know if the fibers are continuous or discontinuous; in other words, are they long or short? Chopped-strand mat is a sheet composed entirely of short fibers. Continuous-strand mat is composed of longer swirled fibers. Easy to shape and quick to saturate with resin, mat is used for building small composite parts for which weight and strength aren’t critical. In larger structures, mat is added among layers of continuous fiber for better wetting-out with resin. Mat offers an economical way to build thickness in a laminate. A chopper gun, which adds short strands of fiber to resin that’s sprayed into a mold, can also build thickness. But chopped or swirled fibers alone create a structure that’s more heavy than strong. Strength comes from fibers that run the length of a structure.
What We Mean When We Say “Fiberglass” It’s common to say, “That’s a fiberglass hull.” Understand the word “fiberglass” is really shorthand for the whole composite. It would be more precise to say, “That’s an FRP hull.” FRP stands for fiber-reinforced polymer, or plastic. (In Britain, the term is GRP: glass-reinforced plastic.) Undertanding the structure of so-called fiberglass hulls means understanding each part of the FRP: the reinforcing fiber and the polymer matrix. |
Two-dimensional sheets of reinforcing fiber may be woven or knitted. The first fiberglass boats in the 1950s were built mostly of fiberglass mat and cloth. Fiberglass cloth is a thin layer that’s composed of finely woven strands. Using cloth alone, it takes many plies to build up a structure that’s thick and strong enough for a hull. Cloth provides a smooth surface and is often used as the first ply in a hull that will be faired.
Woven roving, a loose weave of larger strands that results in a heavier cloth, came on the scene in the 1950s and was the workhorse of composite boatbuilding into the 1970s. With woven roving, thickness in the laminate can be built faster than with cloth, saving labor hours. However, the loose weave leaves lots of space to be filled by excess resin. A resin-rich laminate is heavier than it needs to be to achieve the necessary strength.
Until the mid-1970s, many composite builders equated the strength of the structure with the thickness of the laminate. Boats from that era tend to be heavier than newer boats. Over time, builders and designers became much better at fine-tuning the reinforcing fibers and optimizing strength-to-weight ratios. A light structure depends on minimum amounts of resin matched with the right fibers laid up in the right direction.
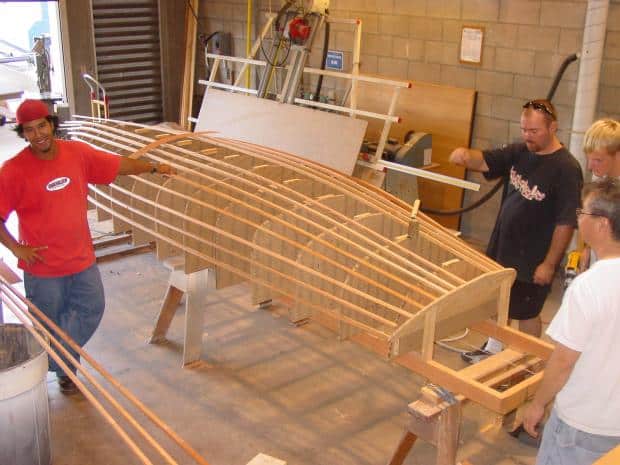
| |In the accompanying series of photos, a team of laminators demonstrate the boatbuilding process known as resin infusion. The first step is to build a plug, the male form from which the builders will take a female mold.| |
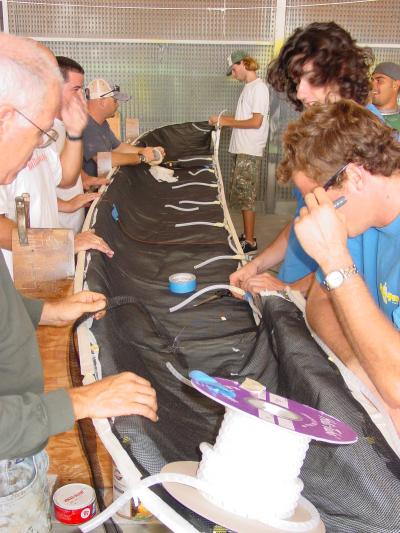
| |Next, they line the mold with sheets of dry fiber reinforcements, then seal it with a plastic bag.| |
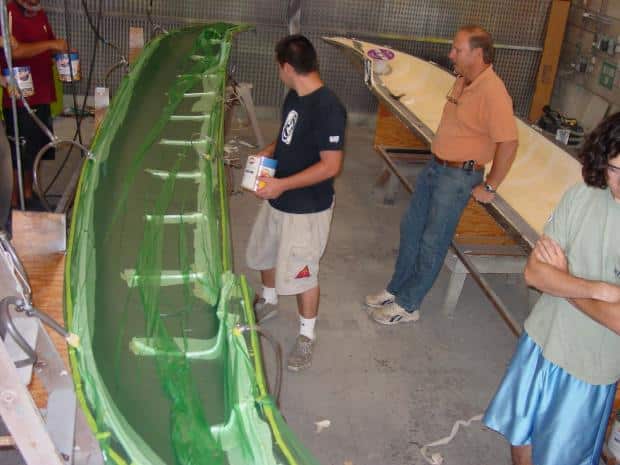
| |_ Finally, they insert hoses into the bag and use vacuum pressure to draw resin through the laminate for an optimum fiber-to-resin ratio._| |
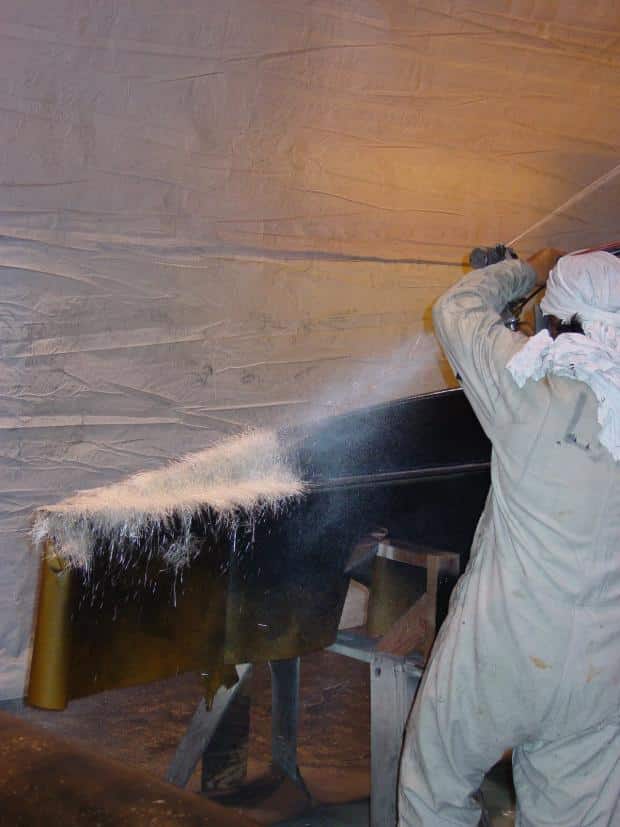
| |A laminator uses a chopper gun, a technique employed in the more traditional contact layup, that builds thickness quickly but at a cost of additional weight.|
In the past 30 years, technology advances have come not just in materials like aramids and carbon but also in how those fibers are arranged in a two-dimensional fabric. For example, knitted fabrics differ from woven roving, whose filaments of equal diameter cross at 90-dgree angles. In knits, the main or structural fibers are stitched together with fine nonstructural filaments, usually polyester. The structural fibers lie flat, not crimped, leaving less space for resin to fill. With knits, builders can get a lot more strength and stiffness per unit thickness.
This reflects a shift in perspective: Whereas earlier builders aimed for thickness (equating thickness with strength), the goal now is maximizing strength. In unidirectional knits, the structural fibers run in one direction that designers can specify for high-load areas, like rig attachment points. In biaxial knits, the structural fibers can be oriented at 45-degree or 90-degree angles for optimal strength in those directions. Further variations—triaxial, quadraxial—provide engineered strength where it’s most needed.
Knitted fabrics often incorporate a layer of mat. For example, 1808 is one popular reinforcing fabric that combines 18-ounce biaxial knit with 8-ounce mat in a single sheet. The mat wets out with resin thoroughly, and the knit provides the directional strength — a smarter way to incorporate engineered strength.
The Resin Matrix
With epoxy and vinylester resins, it’s easy to forget what a revolutionary breakthrough good ol’ polyester was. Though we’ve come to know its shortcomings, polyester remains the keystone of the whole composites industry.
Polyester belongs to a class of chemical compounds called polymers, which can be either thermoplastic or thermosetting. Thermoplastics can be remolded and reshaped every time they’re heated up. PVC and polystyrene are two examples. Once thermosets are cured, the process is irreversible. If you heat a thermoset polymer after it’s been cured, it remains hard and retains its shape. Thermosets include polyester, vinylester, and epoxy. A cured polymer is one in which the molecules are cross-linked with each other. After curing, the product is solid, hard, and chemically resistant.
Polyester resin starts as a solution of carbon, oxygen, and hydrogen groups in a liquid, called a monomer, such as styrene. These chains of molecules link with each other on their own, which is important for builders because the containers of polyester they buy have a limited shelf life; over time, polyester turns from a liquid to a gel and eventually to a solid. Although “polymerization”—the cross-linking of the chemical chain that turns the liquid to a solid—happens naturally in polyester, it doesn’t happen quickly enough on its own to be useful.
When a part is being laid up, the boatbuilder adds a catalyst (often methyl ethyl keytone peroxide, or MEKP) to speed up polymerization to a workable scale of hours and minutes. Other chemicals, called accelerators and inhibitors, can be added to the uncured polyester before or during the process to either speed up or slow down the reaction time. The fact that polyester cures at room temperature is one of its greatest virtues; still, controlling humidity and temperature can help fine-tune the layup. Much of composite boatbuilding is about managing cure times.
In a composite structure, the polymer matrix fulfills three main jobs: It holds the reinforcing fibers in place, acts as a path for transferring loads between the reinforcing fibers, and protects the reinforcing fibers from a brutal environment. Those long reinforcing fibers resist stresses from many directions, but they can’t resist every load from every direction. The matrix must also support against these secondary tension, compression, and shear loads.
Between 1945 and about 1975, orthophthalic polyester was the one and only polymer used in virtually all composite boatbuilding. But several problems were eventually revealed. First, it’s permeable: It lets water in. Not much, but enough to cause problems in the laminate. The chemical mechanism that draws the water into the laminate is called osmosis. The symptom is blistering.
Blistering looks like bubbles in the gelcoat. And while blistering has occurred for as long as polyester has existed, it became a serious problem in the 1970s, as some builders began using resins with different formulas, and boats began breaking out with a pox that was horrendously expensive to fix. In response, builders in the last few decades also work with isophthalic polyester, vinylester, and epoxy resins. Choosing among resin materials is always about balancing properties with cost. As with fibers, savvy builders can mix and match materials to find the right overall blend.
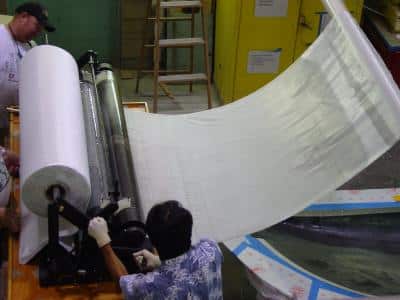
| |A major theme in the 70-year story of composite boatbuilding is the quest for the ideal ratio of resin to fiber. The resin impregnator (above) hangs from a beam in the boat shop and uses a system of rollers to deliver optimally wetted-out sheets of fabric directly to a mold.|
Orthophthalic polyester, or ortho, is the most economical and popular resin used in boatbuilding today. Isophthalic polyester, or iso, has better thermal stability, chemical resistance, and workability. It also resists water permeation much better than ortho. But it’s about 25 percent more expensive. That’s why many cost-conscious builders use iso resin in the gelcoat (the first resin layer that meets seawater) and ortho through the rest of the laminate.
In the 1980s, some builders began experimenting with vinylester resin. Compared to polyester, it resists corrosion, fatigue, and impact much better, and it’s a far better barrier against water intrusion. But it costs about twice as much as ortho. Again, vinylester is sometimes used as an outer barrier coat.
Of all the boatbuilding resins, epoxy has the best properties overall. But it’s about four times as expensive as ortho resins, and it’s used mostly in high-end projects, especially one-off custom boats.
Price in $/pound | |||||
E-glass $.80 – $3.00 | S-glass $8.00 | Aramid (Kevlar-49) $12.00 | Carbon (standard) $15.00 | Carbon (intermediate) $40.00 | Carbon (high mod.) $75-$250 |
Silica-based E-glass is the workhorse of modern boatbuilding, the baseline against which all other fibers are measured. | Another silica-based fiber, it’s more expensive than E-glass, but also stronger, lighter, and less stretchy. | Along with carbon, aramids are a new breed of fiber. The best known, Kevlar, was first developed for tires. | For light weight and stiffness, carbon fiber can’t be beat. It’s costly, but also six times stiffer than E-glass. | Two properties determine carbon quality/cost: “Tow” is the number of filaments; “modulus” gauges stiffness. | The lightest, stiffest composite, but costly and brittle; ideal for high-tech racers, it has limited versatility for cruisers. |
Click here for Part II of this series on boatbuilding.
Editor at large Tim Murphy is the co-author of Fundamentals of Marine Service Technology (ABYC, 2012), from which this story is excerpted.