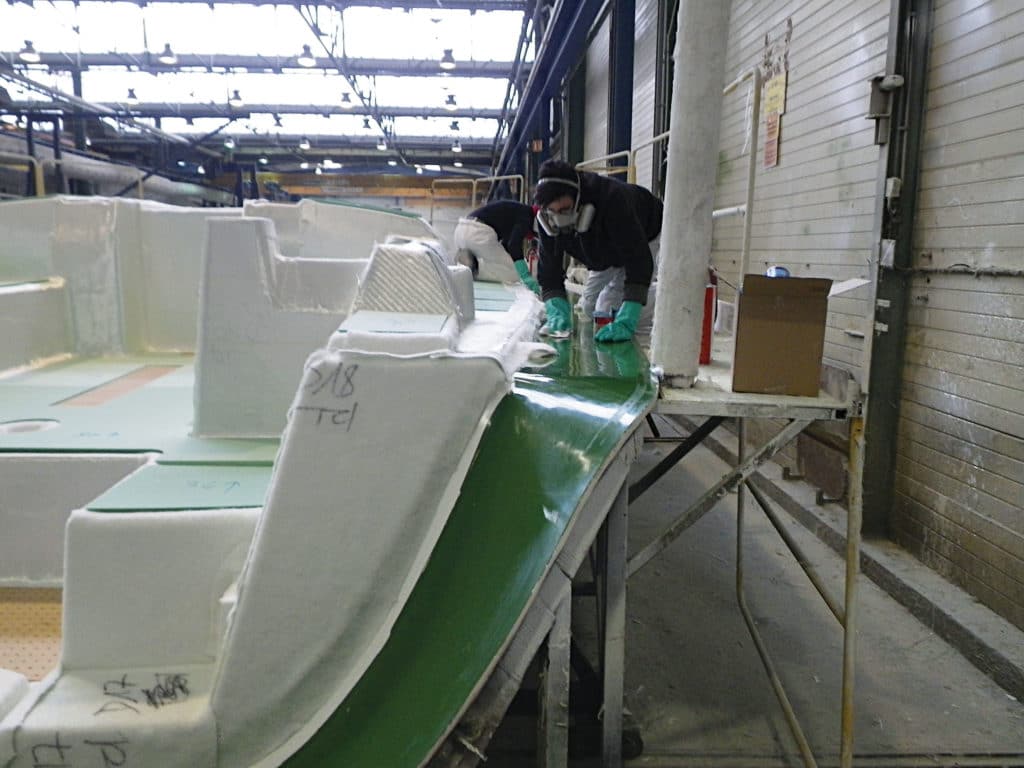
Building a Sandwich
In the first installment of this two-part series (“Decoding the Matrix“), the composite parts we described were solid FRP laminates, also called single-skin parts. They’re composed entirely of fiber and resin. But another critical development in composites technology is sandwich construction. In this concluding article on modern boat construction, we’ll begin there.
Sandwich Construction
In a sandwich, you have an outer skin of reinforced resin, a core in the middle, and then an inner skin of reinforced resin. In engineering terms, the sandwich structure functions like an I-beam: By separating the two skins across a low-density core, it can achieve tremendous strength with remarkably little weight. The resulting structure withstands the bending loads a boat encounters in waves extremely well. The materials used most often to create those low-density cores include end-grain balsa wood, linear PVC foam and cross-linked PVC foam, plus a handful of others.
When Thor Heyerdahl built his raft Kon Tiki to sail across the Pacific Ocean in 1947, the wood he used was balsa. Light and strong, balsa possesses the traits boatbuilders have always sought. But when balsa was first used in composite cores, it was cut with a flat grain. As soon as water got into any part of those structures, it migrated all through the laminate, destroying whole decks and hulls. Today’s balsa-core material is end-grain cut to prevent water migration. End-grain balsa possesses outstanding bond strength and stiffness. It comes in flat sheets, or with serrations and a scrim to conform to complex curves.
Foam made from cross-linked polyvinyl chloride (PVC) has become another industry standard. Trade names include Klegecell and Divinycell. Like balsa, it comes in flat sheets or serrated with a scrim to take complex shapes. Linear PVC (Airex, Core-Cell) has a molecular structure that isn’t connected, so it resists impact better than cross-linked foam.
Other core materials derive their properties from their physical shape. Honeycomb cores, for example, can be made from resins or metals. They tend to be more difficult to bond to the inner and outer skins than balsa or PVC cores. For that reason, they’re used less frequently than balsa or PVC cores in large structural parts like hulls and decks. That said, it’s not uncommon to see honeycombs used to save weight in less-structural components like interior furniture.
No matter which core material is used, the critical component is getting a good bond between the skins and the core. Any voids or delaminations dramatically reduce the strength of the structure. This raises a fundamental distinction that occurs in composite boatbuilding: the difference between primary bonds and secondary bonds. In Part I we discussed the “polymerization” of a resin. That’s the cross-linking of the molecules that make it up. In a primary bond, the molecules link at a chemical level. The only time you can achieve a primary bond in a thermoset polymer is before it’s fully cured. A secondary bond occurs at a physical level, but not at a chemical level. When you apply uncured resin to a cured resin, no molecular cross-linking takes place. Secondary bonds work best when you rough up the surface as you would do with wood; in that case, the curing resin mechanically “hooks” into the porous shapes of the material’s surface. But always remember that a primary bond is far stronger than a secondary bond.
As we consider laminating cores to skins, and as we think about composite techniques more broadly, this distinction between primary and secondary bonds is critical. The best bonds always come when you work with resin that hasn’t fully cured yet.
Composite Layup Techniques
Cured polyester is brittle and heavy. So we never want more of it in the structure than necessary to hold the reinforcing fibers together. A typical low-tech FRP structure might be composed of 40 percent fiber and 60 percent resin. We’d call that a resin-rich structure. More sophisticated methods drive that ratio the other way, toward 70 percent fiber and 30 percent resin. Real-world examples tend to fall somewhere in between. Advances in boatbuilding technology in recent decades have steered toward achieving these better ratios, as well as toward solving polyester’s other problems involving water intrusion, worker safety and environmental concerns.
The bread-and-butter materials of economical marine composite technology are E-glass reinforcement and orthophthalic polyester resin. Other fibers and other resins offer better properties, but they do so at a higher price. Now, as we turn to technique, we can say that hand layup holds the same place in the history and present practice of composite boatbuilding. Hand layup remains the least costly and most common technique, even as more sophisticated methods have come along.
A common hand-layup lamination might go like this: The builder crafts a male plug in the shape of the finished part. The plug may be built of wood and covered with fiberglass cloth and resin, then faired and covered with a release wax and parting film. Or, in some high-production shops, the plug may be robotically sculpted out of foam using a computer-driven CNC cutter. From that male plug a female mold is created, also called the tooling. The majority of today’s boats are built in open female molds. Wax and a release agent go first into the mold, followed by a gelcoat layer of 25 to 30 mils. Next comes a thin layer of mat or cloth, possibly a polyester fabric, whose job is to reduce print-though. (That’s the undesirable pattern of the fiberglass fabric you sometimes see in hulls, especially dark-colored ones.)
Next comes a thin layer of resin, either sprayed or rolled, followed by sheets of reinforcement fabric that have been precut. With grooved rollers or squeegees, the builder then works through the newly laminated section, ensuring that the fabric is thoroughly wetted out. An ideal job is one that achieves a consistent ratio between the fiber and the resin, and leaves no air bubbles or voids.
Specialized tools can speed this basic hand-layup process. Resin can be sprayed and a chopper gun used to deliver short fibers (about 2 inches long) into a lamination. Impregnators are large machines that sometimes hang from gantries over the shop floor and use a system of rollers to deliver wetted-out reinforcements into a mold.
When a cored hull is laid up by hand, the process is similar but with a couple of differences. Because the core material is different from the reinforcing fabric, it’s less flexible across long panel sections and may resist conforming to the complex curves of the mold. The strength of the finished part depends on a complete bond between core and skin. Where a core takes a curve, the spaces formed by the serrations are called kerfs. Any voids will vastly diminish the strength of the finished part. What makes it all harder is that we can’t see behind the core to know whether we have a good bond. A viscous bedding compound can help fill kerfs and other voids. PVC cores can be preheated to make the material better take the new shape. Still, these challenges remain.
This trouble with laminating cores brings us to an important technological development: vacuum bagging. “Dry bagging” is a technique that solves the problem of getting cores to bond thoroughly to the skins. Atmospheric pressure averages around 14.7 pounds per square inch. That’s literally about a ton of pressure for every square foot. And with vacuum bags, that pressure is delivered absolutely evenly across the core.
“Wet-bagging” is a different process altogether. In this case, vacuum lines draw resin through reinforcements that have been laid out and then sealed under a plastic bag. One big advantage of a closed technique like this is that the resin’s fumes can be trapped and collected, separating them from workers and the environment. “Resin infusion,” as it’s also called, describes a technique in which the resin is drawn through the reinforcement under vacuum pressure.
Still other techniques have come along, driven by the twin goals of building better parts and reducing styrene emissions. Another closed-molding technique is called “resin transfer” or “resin injection.” In this case, the reinforcing fiber is laid up between two sides — male and female — of large metal molds. Once the mold is closed and clamped, the resin is applied under positive pressure. An advantage of this technique is that the part comes out of the molds finished on both sides with gelcoat. The disadvantage is that the metal tooling requires a tremendous capital investment. This technique is used by some high-volume yards, where the cost of the tooling can be divided among many boats sold — typically on the order of several dozen or more.
One specialized technique involves pre-impregnated (“prepreg”) reinforcements. The prepreg material requires careful cold storage. Then, at cure time, it needs a very high temperature of at least 140 degrees F. Epoxy is the resin typically used with prepregs. Because the materials are expensive and the shop needs to be specially controlled for heat and humidity, prepregs tend to be used only in high-end custom projects where cutting-edge performance is the goal. Builders using prepreg techniques tend to focus more highly skilled attention on fewer boats at a time.
So there you have it: A brief introduction to the materials and techniques that built the marine industry and nearly every fiberglass boat in it. Moving forward, we hope that in all your projects, or when purchasing a new boat, you’ll think about the properties of the materials involved and ask yourself whether they offer the best combination for the job they do for the vessel they’ve produced.
This article first appeared in the August 2013 issue of Cruising World. Click here to read Part I.